Editor's NoteUnder the sponsorship of the Shanghai Municipal Science and Technology Commission (Project No. 22DZ2304300), The Paper collaborates with World Science to produce popular science reports on award-winning research projects recognized by national and municipal scientific and technological awards.
This article focuses on the first-prize project of the 2020 Shanghai Natural Science Award, “Systematic Research on the Structure and Function of G Protein-Coupled Receptors,” led by Professor Liu Zhijie from the iHuman Institute of ShanghaiTech University.
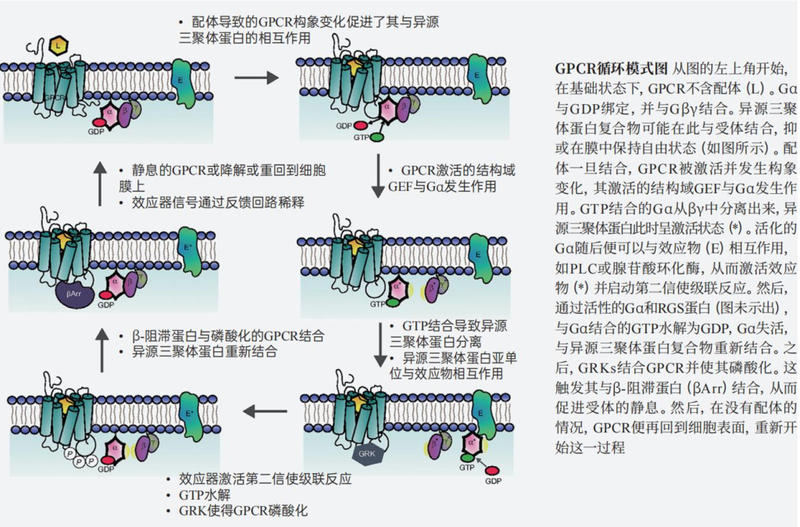
Today, we know that G protein-coupled receptors (GPCRs) are one of the largest and most prevalent families of membrane receptors. There are approximately a thousand genes in the human genome that encode for these receptors, regulating almost all known aspects of human physiological processes, such as sensory modalities including vision, taste, and smell. Consequently, about one-third of the medications approved by the FDA target GPCRs, and this percentage continues to rise.
It is evident that this vast and diverse receptor family is significantly important in the fields of life sciences and pharmaceuticals. With in-depth studies on the structure and function of GPCRs, they have not only become primary targets for drug development but also offer vital insights into understanding the fundamental principles of human physiology.
The Exploration of "Receptors"
Research on receptors plays a crucial role in contemporary biomedical studies; however, it wasn’t until the last thirty to forty years that their existence was widely accepted. For more than a century, how cells perceive and respond to external stimuli has remained a key issue in biology. The “receptor hypothesis,” which posits that receptors span the cell membrane and are capable of sensing and transducing signals, may seem logical today, but when it was first proposed in the early 20th century by British pharmacologist J. N. Langley, it was controversial and contentious. Langley's hypothesis indicated that the structure of receptors has two interconnected functions: first, receptors interact with chemicals or stimuli, most likely through specific modes of binding; second, receptors also act upon intracellular effectors to alter their functions.
Ironically, although Langley’s hypothesis was not recognized by the academic community at the time, his student, Henry Hallett Dale, won the Nobel Prize in 1936 for his research on cholinergic neurotransmission. Now, scientists have identified that acetylcholine receptors are proteins embedded in membranes, with muscarinic receptors (M receptors) being a type of GPCR. For a long time, many scientists indirectly verified the existence of receptors, such as Earl Sutherland.
In the 1940s, Sutherland focused on studying energy metabolism mechanisms in humans during starvation, initiating a series of experiments on how epinephrine and glucagon stimulate the conversion of liver glycogen to glucose. He discovered that these hormones increase the quantity of phosphorylase within the liver via an indirect and complex mechanism. By 1956, he could only demonstrate epinephrine's function within intact liver cells. However, in 1957, he showed that these hormones could activate enzymes in liver homogenates.
Further work by Sutherland and his colleagues revealed that centrifuging liver homogenates rendered the enzymes inactive, and adding hormones to the resultant supernatant would not restore enzyme activity.
However, when hormones were added to the centrifuged cellular debris, they stimulated the formation of a heat-stable factor that activated the enzymes.
This factor was isolated and later identified as 3',5'-cyclic adenosine monophosphate (cAMP), with the enzyme being named adenylate cyclase. Sutherland was awarded the Nobel Prize in Physiology or Medicine in 1971 for this outstanding work. His research demonstrated that epinephrine activates a membrane enzyme—adenylate cyclase—that synthesizes cAMP (a second messenger within cells) and ensures glycogen breakdown through a signaling cascade involving protein kinases.
But how do hormones like epinephrine, neurotransmitters (many of which had already been identified at the time), get recognized by cells?
This led pharmacologists to “forge” the concept of receptors. Receptors were still referred to as receptive substances at that time. Some receptors, especially adrenergic receptors and noradrenergic receptors (such as α- and β-adrenergic receptors), had clearly defined pharmacological characteristics. However, by the late 1960s, receptors still lacked a biochemical entity.
Moreover, many scientific discoveries were not directly related to GPCRs but were indeed closely associated with them. For instance, the Nobel Prize in Physiology or Medicine was awarded in 1967 to scientists who discovered animal phototransduction mechanisms. Today, we know that rhodopsin or photoreceptive proteins belong to the GPCR family, but at the time, the visual system was not connected to cellular signal transduction.
“Perception” and “Seeing” GPCRs
The evidence for the “hypothesis” had become sufficiently compelling, and some scientists began to search for these “invisible yet objectively existing” entities to prove their existence.
In the 1960s, American scientist Martin Rodbell drew an analogy between the computer information processing system and cellular signal transduction processes, refining the second messenger theory and proposing a new operating model requiring three basic components: a discriminator, a transducer, and an amplifier. The receptors on the cell membrane served as discriminators, while downstream activation caused by cAMP could act as an amplifier, with the transducer being the G protein. In 1980, Alfred G. Gilman purified G proteins and discovered that they consist of three subunits: α, β, and γ, hence they are also known as heterotrimeric G proteins.
He further elucidated the mechanism of G proteins: when a ligand binds to a receptor, the heterotrimeric G protein dissociates, exchanging GDP for GTP; the free α-GTP is in an activated state, binding and activating effector proteins to relay signals; when α-GTP hydrolyzes to form α-GDP, it returns to an inactivated state, terminating the signal transduction and causing the heterotrimeric G protein to reassociate, returning the system to a resting state.
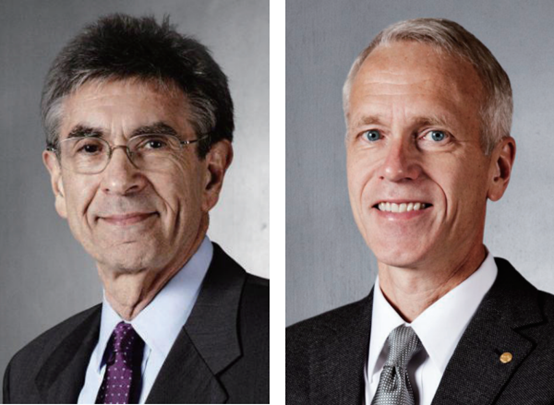
2012 Nobel Prize winners Lefkowitz (left) and Kobilka (right)
Gilman and Rodbell's discoveries revealed the molecular mechanism by which G proteins convert extracellular signals into intracellular signals, ushering in a new era of research in transmembrane cellular signaling. Likewise, they were awarded the Nobel Prize in Physiology or Medicine in 1994 for this outstanding work. Since then, G proteins have begun to gain recognition.
But what about the receptors that serve as "discriminators"? They certainly haven’t been overlooked. Meanwhile, several excellent scientists have also been striving to “perceive” and “see” receptors.
Between 1968 and 1971, American scientist Robert J. Lefkowitz, alongside his students and colleagues, developed radiolabeled ligand binding analysis techniques, using radioactive methods to label glucocorticoids and epinephrine, demonstrating the existence of receptors independent of adenylate cyclase. Through gradual exploration of methods for isolating receptors, Lefkowitz’s team purified the β-adrenergic receptor.
A few years later, after introducing the purified and epinephrine-binding cell surface proteins into cells unresponsive to epinephrine, they found the behavior of these cells was consistent with that of cells possessing adrenergic receptors, providing compelling evidence for the independent existence of cell surface receptors. Additionally, they utilized radiolabeled ligand studies to investigate various factors regulating receptors, discovering previously unknown receptor subtypes, laying the groundwork for developing theories regarding receptor mechanisms of action.
Today, cloning genes may seem like a straightforward task that nearly every biochemistry lab can accomplish. As long as purified adrenergic receptor proteins are available, partial sequence information can be obtained through sequencing, significantly advancing research on adrenergic receptors. However, due to technological limitations at that time, this endeavor was akin to searching for a needle in a haystack. Even after the successful cloning of the rhodopsin gene in 1984, researchers still did not connect photoreceptive receptors and hormone receptors, and due to the extremely low expression levels of hormone receptors, this work became particularly challenging.
In 1986, Brian K. Kobilka joined the project as a research assistant, initially facing significant difficulties. Despite repeated attempts to clone using cDNA libraries, they were unable to achieve the correct results. However, Kobilka proposed a bold idea, attempting to utilize a genomic library to accomplish the cloning task. That year marked a milestone for Lefkowitz’s laboratory, successfully cloning and sequencing the gene encoding the hamster β2-adrenergic receptor protein (β2AR).
Upon thorough investigation of the gene sequence, they were thrilled to discover an astonishing similarity between the β2-adrenergic receptor protein and the amino acid sequence of bovine rhodopsin, both featuring seven transmembrane regions. Lefkowitz and Kobilka astutely inferred that the rhodopsin receptor and β2-adrenergic receptor might belong to the same family, one that potentially encodes many important hormone receptors. Shortly thereafter, cloning experiments of the muscarinic acetylcholine receptor further confirmed this inference.
Based on this, Kobilka and others quickly succeeded in cloning the cDNA encoding the human β2-adrenergic receptor protein, along with the genes for the human β1-adrenergic receptor, human platelet α2-adrenergic receptor, serotonin receptor, and the first orphan receptor. The information and techniques derived from this work laid the foundation for the discovery and cloning of the olfactory receptor superfamily, ultimately earning the Nobel Prize in Physiology or Medicine in 2004 for research in olfactory receptors.
In addition to successfully cloning various receptor genes, Kobilka also undertook the daring task of crystallizing and performing structural analysis on adrenergic receptors. This work was almost deemed impossible at the time due to the low abundance, difficulty in purification, and instability of GPCR proteins, which greatly complicated the crystallization process. Subsequent developments in new technologies proved this viewpoint to be true—Kobilka spent over a decade accomplishing this task.
During his tenure in Lefkowitz's laboratory, Kobilka explored critical regions of GPCRs using methods such as fusion proteins and split proteins. After establishing his laboratory at Stanford University, he continued to refine the functional domain mapping of GPCRs through a series of studies utilizing chimeric receptors and site-directed mutagenesis, and characterized mechanisms of agonist binding and activation through fluorescence spectroscopy, all of which laid a solid foundation for subsequent research into the structure of GPCR proteins.
In 2000, the first high-resolution three-dimensional structure of rhodopsin was published, clearly revealing the seven transmembrane structures of GPCRs and visually demonstrating the interactions between their domains. This research provided Kobilka with invaluable experience, leading him to gradually define the necessary conditions for the purification, dissolution, and stabilization of these molecules, while facing a crisis—his research funding was at risk.
Success comes eventually. In 2007, Kobilka's research burst forth. By co-crystallizing GPCRs with antibodies and inserting lysozyme into the third intracellular loop, he nearly simultaneously obtained the crystals of the β2-adrenergic receptor through both methods. The latter benefited not only from advances in lipid cubic phase technology but also from his prior work exploring the interactions of GPCR structural domains at the molecular level. These results were widely published around the same time in prestigious journals such as Science, Nature, and Nature Methods.
Since then, Kobilka’s laboratory, along with several others, has successfully crystallized about 50 structures of family A GPCRs in the presence of agonists, antagonists, or inverse agonists, and even crystallized the entirely active form of the β2-adrenergic receptor, significantly enhancing our understanding of GPCR workings comprehensively and visually.
For their outstanding work, Kobilka and his former “boss” Lefkowitz shared the 2012 Nobel Prize in Chemistry. Nobel Prizes had previously been awarded multiple times for research relating to GPCRs, encompassing areas like receptors, ligands, senses, and diseases; however, it was this time that a clear and comprehensive understanding of their spatial structures and interactions with G proteins was achieved.
Chinese Scientists Reveal Cannabinoid Receptors Systematically
Deciphering the molecular structure of GPCRs not only enhances our understanding of our physiological structures from a microscopic perspective but also serves as a foundation for developing new drug targets, holding great scientific and practical significance. “Doing research may involve 99.99% failure and 0.01% success, which means you need to endure the 'cold bench,' withstand the test of
Comments