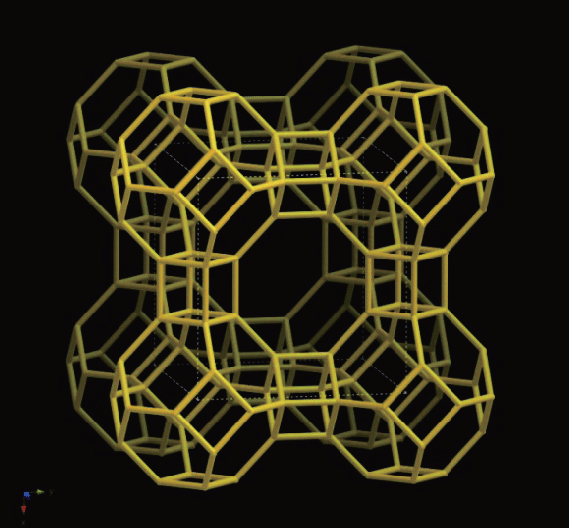
[Editor's Note]Sponsored by the Shanghai Municipal Science and Technology Commission (Project No: 22DZ2304300), The Paper has collaborated with World Science to report on award-winning achievements recognized by national and Shanghai technology awards.
This report focuses on the 2020 National Natural Science Award First Prize project, "Creation and Application of Ordered Mesoporous Polymers and Carbon Materials," led by Professor Zhao Dongyuan, an academician from the Department of Chemistry at Fudan University. On May 26, 2023, at the Shanghai Science and Technology Awards Conference, Zhao Dongyuan was awarded the 2022 Shanghai Science and Technology Pioneer's Award.
Scientific discoveries often happen by chance. When Swedish mineralogist and chemist Alex Cronstedt heated a collected mineral over a flame, he was astonished to observe it bubbling, expanding, and releasing water vapor. After witnessing this intriguing phenomenon, in 1756, the 21st year of the Qianlong era of the Qing dynasty, Cronstedt reported this discovery to the Swedish Academy and named the mineral zeolite—boiling stone—what a vivid name!
However, Cronstedt was not aware of the underlying reasons for the phenomena he observed at the time. Research on zeolites fell into a long silence after him, and it wasn't until over a century later that researchers demonstrated that the water loss phenomenon Cronstedt had noted was reversible, meaning that zeolites could reabsorb water after dehydration, cycling continuously. Additionally, reports indicated that zeolites possess ion-exchange properties, where the metal ions on the zeolite can be replaced by metal ions in the solution.
The "Truth" of Zeolites
Modern scientific research has revealed that zeolites are a class of porous crystalline materials composed of aluminosilicates, featuring pore channels and cavities of molecular sizes ranging from 0.3 nm to 1.5 nm. In the early 20th century, the commercial value of zeolites was uncovered for softening hard water by removing excessive calcium and magnesium ions, which are still added to laundry detergents today to improve washing performance. In 1925, the phenomenon of zeolite separating gas molecules was first reported, demonstrating that zeolite crystals could separate gas molecules based on size after removing water from their pores. By 1932, this property of zeolites was termed "molecular sieve" action, and it acquired a new name—molecular sieve—which first appeared in scientific publications. Inspired by this, British chemist Richard Barrer began an in-depth study of zeolite molecular sieve's gas adsorption properties and started the artificial synthesis of zeolite molecular sieves. In 1948, he proposed a concept to synthesize zeolite molecular sieves under simulated geological conditions—that is, high temperatures and the pressure generated by boiling water. Guided by this idea, they successfully achieved the synthesis of synthetic zeolite molecular sieves with entirely new structures that do not occur in nature.
This success opened the door to hydrothermal synthesis of zeolite molecular sieves. To this day, hydrothermal reactions remain a vital method for laboratory synthesis and industrial production of zeolite molecular sieves.
The Era of Synthetic Zeolites
As the 1950s approached, the commercial value of zeolite molecular sieves became increasingly apparent. Researchers from the industrial sector rapidly joined the research on zeolite molecular sieves. A notable representative was Robert Milton from the United Carbon Company’s Linde Air Products Division. Through exploration, Milton adopted new raw materials and successfully synthesized three new types of zeolite molecular sieves—types A, B, and X—under gentler conditions than Barrer's methods. Since their commercialization as industrial adsorbents in 1954, A-type (named Linde Type A, LTA) and X-type zeolite molecular sieves continue to hold significant positions in the adsorbent and catalyst markets.
Due to these significant contributions, Barrer and Milton are regarded as the pioneers of zeolite molecular sieve chemistry. Under their leadership, the artificial synthesis of zeolite molecular sieves has garnered extensive research, revealing various zeolite molecular sieve framework topologies.
To date, the International Zeolite Association (IZA) website lists 247 types of framework topology for molecular sieves. Below, Figure 1 displays the LTA structure of the A-type sieve, while Figure 2 presents the Faujasite (FAU) structure of the X-type molecular sieve.
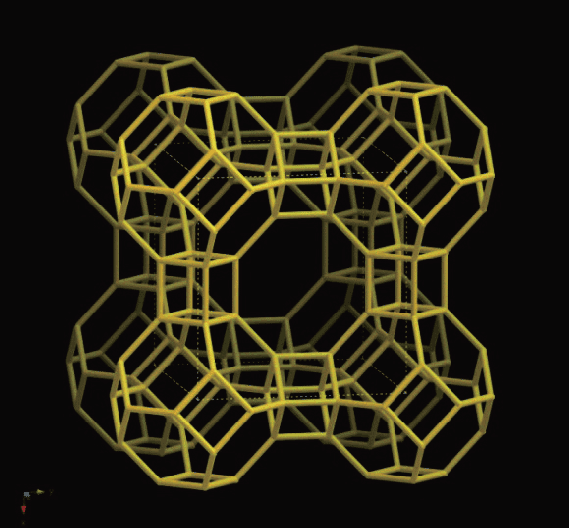
LTA framework topology
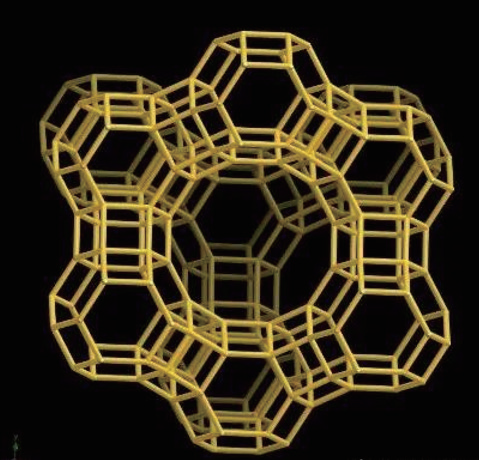
FAU framework topology
Starting from 1959, United Carbon Company promoted the large-scale application of synthetic zeolite molecular sieves in separating petroleum products, using calcium-containing A-type zeolite molecular sieves to adsorb and separate straight-chain normal alkanes with five or six carbon atoms from gasoline, while retaining branched isomers to enhance the gasoline's octane rating. At the same time, the catalytic properties of zeolite molecular sieves began to gain attention.
Researchers quickly discovered that zeolite molecular sieves have an extremely high specific surface area (often reaching hundreds of square meters per gram), strong adsorption capabilities, numerous catalytic active sites, stable structures, thermal stability, hydrothermal stability, chemical corrosion resistance, and that their adsorption properties can be easily adjusted from hydrophilic to hydrophobic. The dimensions of the pores and cavities match many valuable molecules, and their complex pore structures are favorable for achieving specific reactions (shape-selective catalysis).
Notably, the representative zeolite molecular sieve catalysts include the Y-type molecular sieve synthesized by United Carbon Company researchers in 1954 and the Socony Mobil-5 zeolite (ZSM-5) synthesized by Mobil Oil researchers in 1963. The Y-type molecular sieve, which has a framework structure similar to the X-type sieve (FAU), serves as a commonly used strong acidic catalyst in oil catalytic cracking, hydrogenation cracking, and isomerization reactions. ZSM-5 is characterized as a high-silica content zeolite molecular sieve with an MFI (Mobil-type Five) structure (Figure 3) and exhibits excellent shape-selective catalytic performance in reactions such as paraffin dehydrogenation and methanol conversion.
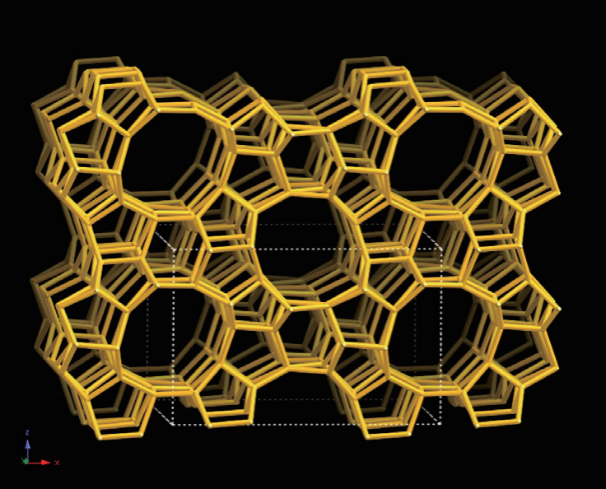
MFI framework topology
Thus, zeolite molecular sieves gradually replaced traditional amorphous silica and alumina catalysts to become significant catalysts in the petroleum refining sector, profoundly impacting the petrochemical industry and becoming one of the most widely used catalysts in industrial fields today. Starting in the 1970s, as petroleum resources became increasingly scarce, deep processing of larger molecules, such as heavy oil, became essential. However, the smaller pore size characteristics of zeolite molecular sieves limited their applications in this regard, prompting researchers to shift their focus to the development of porous materials with larger pore sizes. The drums of research began to beat, and positive news continued to emerge. In 1982, aluminum phosphate molecular sieve AlPO4-8, containing a 14-membered ring, came forth, breaking the iron rule that the largest pore of zeolite molecular sieves could only be composed of 12 atoms. In 1988, Virginia Polytechnic Institute's molecular sieve VPI-5 refreshed this record, achieving a pore consisting of 18 atoms. In 1991, the 20-membered ring pore of the gallium phosphate molecular sieve Cloverite again set a new record.
According to the International Union of Pure and Applied Chemistry (IUPAC), materials with pore sizes smaller than 2 nm are classified as microporous materials, while those larger than 50 nm are termed macroporous materials. Meanwhile, materials with pore sizes between 2 nm and 50 nm are referred to as mesoporous materials. While significant progress was made in the field of synthetic zeolite molecular sieves during the 1980s, it still lingered in the realm of microporous materials.
At the same time, layered materials were also held in high regard. Layered materials represented by montmorillonite, phosphates, and double hydroxides can insert metal ion oligomers or quaternary ammonium salts as guest species into their interlayers through ion exchange, resulting in enlarged interlayer spacing, a process known as "pillar supporting." Although these pillar-supported layered materials exhibit pore sizes larger than micropores, their arrangements are chaotic, leading to broad pore size distributions. Consequently, molecules struggle to transport smoothly through the pore channels or achieve selective adsorption, greatly limiting their application in catalysis. The urgent need arose to develop ordered mesoporous materials with regular pore channels.
The Era of Mesoporous Molecular Sieves
In 1991, researchers from Mobil Oil published the first report on the synthesis of ordered mesoporous molecular sieve materials in the journal Nature. By using a quaternary ammonium cationic surfactant, cetyltrimethylammonium bromide, as a template, along with aluminum oxide, silicate, and silica as reaction precursors, they synthesized an ordered mesoporous silicon (aluminum) molecular sieve material through a hydrothermal reaction and subsequent calcination process to remove the template. The resulting product featured a uniformly ordered hexagonal pore arrangement (Figure 4) resembling a "honeycomb" structure, with a specific surface area exceeding 1000 m²/g and adjustable pore diameters between 1.5 nm and 10 nm.
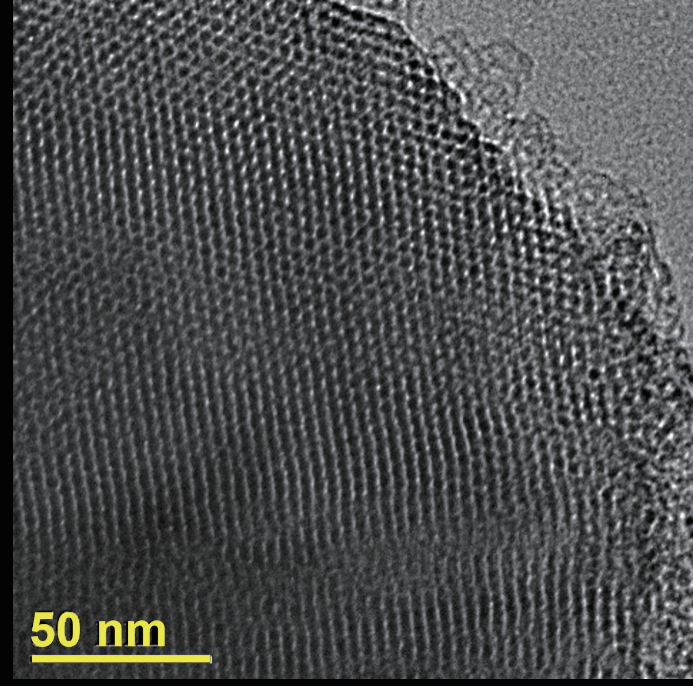
Transmission electron microscope image of MCM-41
They named this material Mobil Composition of Matter-41 (MCM-41). Though considered a porous material, this ordered mesoporous molecular sieve differs from traditional microporous zeolite molecular sieves, as its pore walls are amorphous rather than crystalline. More importantly, this report introduced the concept that the liquid crystal structures formed by surfactants in solution are crucial to constructing ordered mesoporous structures, a mechanism that later guided the synthesis of many ordered mesoporous materials, known as the "liquid crystal template mechanism." Since the surfactants used were "flexible" molecules that dissolve in solution, this type of template was referred to as a "soft template," making the corresponding "liquid crystal template mechanism" akin to the "soft template mechanism." The emergence of MCM-41 sparked a wave of interest in the research of ordered mesoporous materials, quickly becoming a hot topic across several fields, including nanomaterials chemistry, molecular sieve chemistry, and catalytic chemistry.
However, perfection is elusive. MCM-41 has some drawbacks, particularly its relatively thin pore walls and poor hydrothermal stability, which are linked to the use of ionic surfactants as templates. These limitations spurred researchers to explore new methods for synthesizing ordered mesoporous silica molecular sieves.
Professor Zhao Dongyuan and Mesoporous Molecular Sieves
In 1998, a new breakthrough occurred. An article published in Science by Zhao Dongyuan and Galen Stucky from the University of California, Santa Barbara, reported the use of a nonionic surfactant, polyethylene oxide-polypropylene oxide-polyethylene oxide triblock copolymer, as a soft template in an acidic medium, with a water-soluble silicon source as the precursor. They synthesized an ordered mesoporous silica molecular sieve with a hexagonal pore "honeycomb" structure similar to MCM-41 through a hydrothermal reaction. The new method achieved thicker pore walls, and interconnected micropores within the pore walls, resulting in enhanced thermal stability compared to MCM-41. Additionally, the pore size could be expanded up to 30 nm. This new ordered mesoporous silica molecular sieve was named Santa Barbara Amorphous-15 (SBA-15). As a "rising star" in the field of ordered mesoporous molecular sieves, SBA-15's emergence triggered a research boom, both in ordered mesoporous materials and across the broader realm of nanomaterials. At the end of 1998, Professor Zhao Dongyuan joined Fudan University to continue research into ordered mesoporous materials, leading to a series of ordered mesoporous silica materials named FDU.
As ordered mesoporous materials flourished, researchers also delved into alternative approaches for synthesizing them, namely hard template methods. The so-called hard template method involves using materials with a pre-existing stable ordered mesoporous structure as the template framework, filling the gaps in the framework with precursor materials, and then removing the framework material to synthesize a different ordered mesoporous structured material. This strategy closely resembles the casting process of traditional metallic components and is thus dubbed "nano